Commentary
What is the future for Scapholunate interosseous ligament reconstruction?
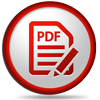
Amelia Carr1, Saso Ivanovski1, Randy Bindra2 and Cedryck Vaquette1*
1The University of Queensland, School of Dentistry, Herston, Queensland, Australia
2Griffith Health Institute, Griffith University, Gold Coast, Australia
*Address for Correspondence: Dr. Cedryck Vaquette, The University of Queensland, School of Dentistry, Herston, Queensland, Australia, Email: c.vaquette@uq.edu.au
Dates: Submitted: 12 December 2018; Approved: 28 December 2018; Published: 31 December 2018
How to cite this article: Carr A, Ivanovski S, Bindra R, Vaquette C. What is the future for Scapholunate interosseous ligament reconstruction? Arch Clin Exp Orthop. 2018; 2: 004-007. DOI: 10.29328/journal.aceo.1001004
Copyright Licence: © 2018 Carr A, et al. This is an open access article distributed under the Creative Commons Attribution License, which permits unrestricted use, distribution, and reproduction in any medium, provided the original work is properly cited.
Keywords: Scapholunate interosseous ligament; Finite element analysis; 3D-printing
Abstract
This opinion paper provides a summary of the current reconstructive surgical techniques for the scapholunate interosseous ligament (SLIL) and critically highlights their benefits and shortcomings. Due to limited success with direct repair of the ligament, current practice focuses on achieving biomechanical stabilization and does not allow for tissue regeneration to occur. In addition, the biomechanical behaviour of the ligament is still poorly described and understood, resulting in a very large variation in published mechanical parameters. Therefore, a thorough understanding of the biomechanics of the joint, via both experimental testing and numerical modelling is necessary for enabling the design of the next generation of implants in order to address mechanical stabilisation and regeneration.
Commentary
The scapholunate interosseous ligament is an anatomically complex tissue displaying a C-shape and is located between the scaphoid and lunate bones in the wrist and is responsible for the stabilization of the joint [1]. Damage to the scapholunate interosseous ligament (SLIL) is the most common ligament injury in the wrist with a particularly high incidence rate in young active individuals engaging in contact sports [2]. Healing potential of complete tear of the SLIL is essentially non-existent due to its poor vascularization; therefore surgical intervention is necessary to maintain wrist stability and motion [3,4]. In fact, current treatment options for the management of SLIL injuries are unable to restore wrist mobility and hand function. In advanced cases of wrist instability or failed ligament reconstruction, fusion of carpal bones is performed, trading off wrist mobility for stability and pain relief. Scapho-lunate arthrodesis directly addresses the unstable joint but has been largely abandoned because of low fusion rates [5].
In an attempt to replace the SLIL and maintain a certain level of mobility in the wrist, some techniques use autologous bone-ligament-bone grafts harvested from either the foot or the wrist [6-11]. In this approach, anatomical and compositional similarities to the SLIL are desirable features. However, these grafts are limited by inadequate mechanical properties, poor bony fixation and donor morbidity. The use of tendon grafts routed through drill holes in the scaphoid and lunate have also been investigated, however the long term outcome is not known [12,13].
As seen above, there exists a plethora of reconstructive procedures and none has emerged as the gold standard surgical solution. The major drawback with all current reconstructive techniques is the lack of biomechanical similarity to the natural tissue. Current techniques involve either under-engineered constructs trying to mimic the natural tissue but neglecting stability, or over-engineered constructs trying to stabilize the joint but neglecting tissue mimicry. Therefore, these techniques involve long recovery times, limited weight bearing and/or motion, and none are currently able to restore the original kinematics of the wrist.
There is a lack of in-depth understanding of the biomechanical properties of the SLIL with a limited number of studies performed to investigate these features. This could be related to the complexity of the relationship between the SLIL and the surrounding extrinsic wrist ligaments, making it difficult to isolate the SLIL for anatomic study. Another difficulty is the limited availability of young cadaveric samples for investigation.
Additionally, there appears to be a great variation in the measured mechanical parameters which could be related to 1) different biomechanical testing protocols and 2) the utilization of already damaged or degenerated human SLIL cadaveric specimen, 3) inherent dimensional, anatomical and biomechanical differences between humans. Consequently, basic biomechanical properties, such as the strain or force experienced by the ligament during flexion, extension or even in the neutral position cannot be conclusively answered. Therefore, there is a clear need for better characterization of the SLIL using both direct measurements from larger patient cohorts and numerical modelling in order to decipher the true biomechanical behaviour of the SLIL.
The future of SLIL reconstruction relies on a more profound understanding of its biomechanical behaviour in order to design the next generation of implants. Recently, the utilization of tissue engineering techniques, such as decellularization and the utilization of synthetic materials, have been explored for SLIL reconstruction [14]. The successful Ligament Augmentation and Reconstruction System (LARS) is a synthetic braided polyester ligament replacement device and has been trialed as a SLIL substitute [15]. This technique reduced the scapholunate gap and corrected unnatural flexion and extension, however there were concerns regarding the amount of fibrous ingrowth, ligament slippage from the bone tunnels, and wear of the artificial ligament [15]. Although these studies were limited to assessing the biomechanical stability of the implants in a cadaveric setting, they provide a shift in the current strategies which mostly involve natural tissues.
Our group is working towards the development of a personalized synthetic scaffold for SLIL reconstruction fabricated via an additive manufacturing method as previously reported for the scaphoid and lunate bone only [16]. However, the fabrication of a multi-compartment Bone-Ligament-Bone scaffold using additive manufacturing remains elusive. The technology utilizing fused deposition modelling (FDM), whereby a polymer is molten and extruded into a thin filament, will enable the manufacturing, in a continuous manner, of a highly porous medical grade biodegradable scaffold as previously reported by our group albeit for different applications [17-22]. This porous custom 3D-printed Bone-Ligament-Bone scaffold will be able to accommodate variations in both the tissue geometry and the biomechanical requirement while providing a scaffold to enable tissue regeneration. As the printing is performed in continuous manner, a porous interface between the bone and ligament compartments is maintained which is essential for ensuring the proper integration and anchorage of the newly formed ligament in the bone which is a challenge in the field. This approach combines clinical imaging (MRI or CT scan), finite element analysis (FEA), which has been previously used to model physiological deformation by applying load in the tenths of newton range [23]. The FEA is then coupled with additive manufacturing to develop a 3D-printed scaffold where the geometry is patient-customized and the mechanical properties are patient specific. We believe that the personalized approach combining these technologies focused on SLIL regeneration will form a platform solution that may be adapted to other areas of ligament injury.
References
- Berger RA. The gross and histologic anatomy of the scapholunate interosseous ligament. J Hand Surg Am. 1996: 21: 170–178. Ref.: https://goo.gl/Mv8ocm
- Avery DM 3rd, Rodner CM, Edgar CM. Sports-related wrist and hand injuries: A review. J Orthop Surg Res. 2016; 11: 1–15. Ref.: https://goo.gl/jnGYRC
- Izadpanah A, Kakar SS. Acute Scapholunate Ligament Injuries: Current Concepts. Oper Tech Sports Med. 2016; 24: 108–116. Ref.: https://goo.gl/jCMsmQ
- Nikolopoulos FV, Apergis EP, Poulilios AD, Papagelopoulos PJ, Zoubos AV, et al. Biomechanical properties of the scapholunate ligament and the importance of its portions in the capitate intrusion injury. Clin Biomech. 2011: 26: 819–823. Ref.: https://goo.gl/DEYnws
- Kitay A, Wolfe SW. Scapholunate instability: Current concepts in diagnosis and management. J Hand Surg Am. 2008; 33: 998-1013. Ref.: https://goo.gl/Ceim2t
- Hofstede DJ, Ritt MJPF, Bos KE. Tarsal autografts for reconstruction of the scapholunate interosseous ligament: A biomechanical study, J Hand Surg Am. 1999; 24: 968-976. Ref.: https://goo.gl/XwCVco
- Müller M, Reik M, Sauerbier M, Germann G. A new bone-ligament-bone autograft from the plantar plates of the toes and its potential use in scapholunate reconstruction: An anatomical study. Ann Plast Surg. 2008; 61: 463–467. Ref.: https://goo.gl/cGVtzw
- Shin SS, Moore DC, McGovern RD, Weiss AP. Scapholunate ligament reconstruction using a bone-retinaculum-bone autograft: A biomechanic and histologic study. J Hand Surg Am. 1998; 23: 216–221. Ref.: https://goo.gl/shZVaA
- Svoboda SJ, Eglseder WA Jr, Belkoff SM. Autografts from the foot for reconstruction of the scapholunate interosseous ligament. J Hand Surg Am. 1995; 20: 980–985. Ref.: https://goo.gl/7Yg91w
- Weiss AP. Scapholunate Ligament Reconstruction Using a Bone-Retinaculum-Bone Autograft. J Hand Surg Am. 1998; 23A: 261–264. Ref.: https://goo.gl/VW4Eas
- Harvey EJ, Hanel D, Knight JB, Tencer AF. Autograft replacements for the scapholunate ligament: A biomechanical comparison of hand-based autografts. J Hand Surg Am. 1999; 24: 963-967. Ref.: https://goo.gl/amJypm
- Brunelli GA, Brunelli GR. A new technique to correct carpal instability with scaphoid rotary subluxation: a preliminary report. J Hand Surg Am. 1995; 20: S82-85. Ref.: https://goo.gl/CuWnw1
- McGrath T, Zivaljevic N. New Technique for Anatomic Reconstruction of the Scapholunate Ligament with Tendon Graft and Swive Lock Anchor Fixation: A Biomechanical Cadaveric Study: Not a clinical study. J Hand Surg Am. 2012; 37: 13. Ref.: https://goo.gl/bVDhbN
- Endress R, Woon CY, Farnebo SJ, Behn A, Bronstein J, et al. Tissue-engineered collateral ligament composite allografts for scapholunate ligament reconstruction: an experimental study. J Hand Surg Am. 2012; 37: 1529-1537. Ref.: https://goo.gl/FkFoo1
- Eng K, Wagels M, Tham SK. Cadaveric scapholunate reconstruction using the ligament augmentation and reconstruction system. J Wrist Surg. 2014; 3: 192-197. Ref.: https://goo.gl/5TUhJw
- Gittard SD, Narayan RJ, Lusk J, Morel P, Stockmans F, et al. Rapid prototyping of scaphoid and lunate bones. Biotechnol J. 2009; 4: 129-134. Ref.: https://goo.gl/nQM159
- Costa PF, Vaquette C, Baldwin J, Chhaya M, Gomes ME, et al. Biofabrication of customized bone grafts by combination of additive manufacturing and bioreactor knowhow, Biofabrication. 2014; 6: 035006. Ref.: https://goo.gl/dDJ7Ki
- Costa PF, Vaquette C, Zhang Q, Reis RL, Ivanovski S, et al. Advanced tissue engineering scaffold design for regeneration of the complex hierarchical periodontal structure. J Clin Periodontol. 2014; 41: 283-294. Ref.: https://goo.gl/LLWF5Z
- Hamlet SM, Vaquette C, Shah A, Hutmacher DW3, Ivanovski S. 3-Dimensional functionalized polycaprolactone-hyaluronic acid hydrogel constructs for bone tissue engineering. J Clin Periodontol. 2017; 44: 428-437. Ref.: https://goo.gl/U4SZ88
- Lee SJ, Lee D, Yoon TR, Kim HK, Jo HH, et al. Surface Modification of 3D Printed Polycaprolactone Constructs via a Solvent Treatment: Impact on Physical and Osteogenic Properties. ACS Biomater Sci Eng. 2019; 5: 318–328. Ref.: https://goo.gl/hwgrd2
- Sudheesh Kumar PT, Hashimi S, Saifzadeh S, Ivanovski S, Vaquette C. Additively manufactured biphasic construct loaded with BMP-2 for vertical bone regeneration: A pilot study in rabbit. Mater Sci Eng C Mater Biol Appl. 2018; 92: 554-564. Ref.: https://goo.gl/GbFPrJ
- Vaquette C, Fan W, Xiao Y, Hamlet S, Hutmacher DW, et al. A biphasic scaffold design combined with cell sheet technology for simultaneous regeneration of alveolar bone/periodontal ligament complex. Biomaterials. 2012; 33: 5560-5573. Ref.: https://goo.gl/rdCczh
- Alonso-Rasgado T, Zhang QH, Jimenez-Cruz D, Bailey C, Pinder E, et al. Evaluation of the performance of three tenodesis techniques for the treatment of scapholunate instability: flexion-extension and radial-ulnar deviation. Med Biol Eng Comput. 2018; 56: 1091-1105. Ref.: https://goo.gl/j6pJ98